
From Dust to Stars: A Guide to The Process of Star Formation
Last Updated: December 29, 2022
Have you ever gazed up at the night sky and marveled at the twinkling stars above? But have you ever wondered how these celestial bodies come to be? What is their life cycle, and what happens when they eventually reach their end? Join us as we explore the fascinating process of star formation and the mysteries that surround the death and rebirth of these awe-inspiring objects.
From the birth of a star to its eventual demise, we’ll delve into the science behind it all and consider the possibility of life beyond a star’s death.
How do we study stars
Stars continue to fascinate us after thousands of years. To the naked eye, many appear white in the night sky, though you may find some red ones, ones that appear golden or orange, or even ones that have a blue tinge.
Some seem smaller or bigger, and while this is often impacted by how far away the star is from us, we do know that stars come in a variety of sizes. Telescopes, especially ones that can detect other electromagnetic wavelengths than the range our eyes can detect, have helped us to better understand star formation by studying stars in different phases of their lifecycle.
Related article: From Birth to Death: The Lifecycle of Our Sun
The James Webb Telescope is the newest technology to continue the work, with an over 21-foot (6.5 meter) telescope capable of detecting light in the gold-colored visible to the mid-infrared. One of its main focuses is studying the early universe when some of the earliest stars and galaxies were forming.
Now that we understand the history of studying stars, let’s go through the life cycle of a star.
Early Life: Star Formation/ Protostar
Stars form in clouds of gas and dust called nebulae or stellar nurseries that can form anywhere between a few dozen to thousands of stars. As particles of dust are attracted to each other, blobs or knots of gas and dust begin to form and spin around each other. Once they have enough mass and speed, they will begin to collapse into themselves and heat up at the center.
This hot core is a protostar, waiting to begin life as a star as soon as it is hot and dense enough for nuclear fusion to occur. It would take a collection of gas and dust a hundred times the size of our solar system to create a star like our Sun 864,400 miles (1,391,000 kilometers) across. Any remaining dust can become other parts of a solar system, such as planets, asteroids, or comets; but they also may remain as dust.
If the protostar cannot acquire enough mass, a brown dwarf can form, a substellar object that is unable to achieve and sustain hydrogen fusion in its core thereby leaving an object that is not a planet, but neither a star. While these used to only be theoretical, we now have evidence to support the idea that there is a brown dwarf for every six stars.

Nebulae are rich in star-making material. They really are the cosmic nurseries of our universe.
Mid-life: Main Sequence Stars
A star is born once the hot core at the center of a blob/ knot of gas and dust becomes heavy and hot enough for hydrogen atoms to overcome their natural repulsive tendencies and fuse into one Helium atom, releasing an enormous amount of energy.
Quick aside: Nuclear fusion is the opposite of nuclear fission, splitting an atom, which we have been able to harness to create nuclear weapons and nuclear power plants. The goal of nuclear fusion as a renewable source of energy has long been sought after and while nuclear electric plants are a viable energy source, they have yet to create more energy as output than was put in and they create a lot of waste that remains radioactive for decades, neither of which are ideal for a sustainable renewable resource. This hurdle has just been surpassed on December 5th, 2022 by the Lawrence Livermore National Laboratory’s National Ignition Facility in California. A 192-beam laser was used to heat a tiny amount of hydrogen atoms in a capsule no bigger than a peppercorn until the atoms heated and compressed, ultimately forcing the atoms to fuse and release energy, resulting in an inputted 2.05 megajoules (MJ) of energy which produced 3.15 MJ of fusion energy output, a net gain of 1.1 MJ! We remain cautiously optimistic about these results, as they could be the start of a new era in nuclear energy, but there is still an immense amount of work to be done to make this a sustainable, safe renewable resource. But now, let’s get back to stellar formation.
The outflow of energy from the fusion occurring in the heart of the star provides adequate pressure to prevent the star from collapsing in on itself. While not a perfect analogy, think of a balloon. It’s a balancing act of increasing the air pressure inside the rubber to form a solid shape. Too little air and the balloon goes limp. Too much air and it pops, but there are many sizes in between as long as everything is kept in balance. Once nuclear fusion occurs, the star contracts a little and becomes stable, as it will remain for millions or even billions of years.
Once a star is born, it is known as a Main Sequence star and will remain so until it begins to die. There are many different types of Main Sequence stars in a wide range of colors, sizes, levels of brightness, and temperatures. As shown by the Hertzsprung-Russel Diagram, there is a general trend in age, size, and color.
Despite what we normally see here on Earth, red stars are cooler. They are still very hot, but cool in relation to other stars. The smallest and coolest stars are typically red with red dwarfs being as small as 10% of the mass of our Sun and emitting only 0.01% of our Sun’s energy at only 3,000-4,000 degrees Kelvin (4,940-6,740 F or 2,727-3,727 C). They are numerous and typically old.
Orange and yellow stars like our Sun are typically medium/ average in both size and temperature. Blue and blue-white stars are the hottest. While we typically think of red as hot and blue as cold, we do see this on Earth sometimes. After the flame is blown out, the wick of the candle stays white until it cools to red and then black. When we look at welding or bunsen burners, blue and white flames are the hottest while yellow or red flames are cooler, again, still hot and can still cause damage, but lower on the scale.
(Blue) White stars are typically larger, brighter, and hotter. These include the hypergiants in the far extreme. They are immensely hot with surface temperatures at more than 30,000 K, emitting thousands of times more energy than the Sun, and are at least 100 times more massive than the Sun, but often extremely young, only a few million years old.
The End of Life: The Death of a Star
A star’s life is generally dictated by its size. The larger a star is, the shorter its life will be, though billions of years is a typical lifetime for all but the most massive stars.
As hydrogen is fused to make helium, its supply of hydrogen dwindles, and slowly the core uses up all of its hydrogen. The star becomes heavier (since helium atoms are heavier than hydrogen) and it becomes harder for it to maintain the balance crucial to being a star. The core begins to collapse in on itself, increasing temperature, and then fusing what hydrogen is left outside the core. The outer layers of the star push outward, causing it to expand and cool into a red giant or red supergiant if it is massive enough.
If a star is sufficiently massive, the collapsing core can become hot enough to fuse heavier and heavier elements, forming the elements that will make up future planets and even life forms. But as heavier and heavier elements are formed, the nuclear reactions will become increasingly unstable, burning furiously, dying down, and then burning furiously again, causing the star to pulse and throw off its outer layers, leading to a variety of options.
The end of an average star (up to about 1.4 times the mass of our Sun)
- These will continue ejecting layers into a planetary nova as helium is fused into carbon and the core ultimately collapses, leaving behind a White Dwarf, essentially an immensely hot cinder of the stellar fire it was.
- White Dwarfs are about the size of the Earth, but contain the mass of a star. Even a teaspoon of one could weigh as much as an elephant– 5.5 tons. Quantum mechanics explain that the pressure from the electrons of the surviving atoms keep the star from collapsing further. This creates a contradictory phenomenon in which the smaller a white dwarf is in diameter, the larger it is in mass as the more massive the stellar core, the denser the white dwarf.
- They are very faint because they are so small and since they no longer have an active nuclear reaction, they will slowly cool down and fade away to what is called a black dwarf though we cannot detect them since they emit the same temperature as the microwave background of the universe. That, combined with the current understanding of the oldest known stars, means there are no known black dwarfs today.
Some white dwarfs will become planetary novae if they are in a binary or multiple star system as they may drag matter from the outer layers of the nearby star onto its surface layer, causing a burst of nuclear fusion when enough matter is gathered, brightening substantially and expelling material.
This cycle can happen multiple times, especially for the bigger stars within this category, eventually leading to a supernova if they get large enough.
The end of a large star (between 1.4 and 3 times the mass of our Sun)
- The collapse of the core continues, fusing together until electrons and protons fuse to form neutrons, creating a neutron star. These are incredibly dense (similar to the density of an atomic nucleus), creating immense gravity due to their immense mass in a tiny volume.
- Similar to White dwarfs, a neutron star can also strip matter of companion stars if it is in a binary or multiple star system.
Neutron stars have powerful magnetic fields which can produce powerful beams of radiation around their magnetic poles due to accelerating atomic particles.
Like searchlights, these beams of radiation sweep around as the star rotates and if they point toward Earth, we observe regular pulses of radiation, leading to the term pulsars for these neutron stars.
The end of an extra-large star (larger than 3 times the mass of our Sun)
The end of an Hypergiants (stars more than 8 times the mass of our Sun)
- The core collapses and explodes in a supernova.
- Once it has fused enough elements to create iron, nuclear fusion can no longer continue as fusions of elements higher than iron consume more energy than they create. Since it can no longer support its own mass, the iron core collapses, shrinking from roughly 5000 miles across to just a dozen in just seconds, shooting the temperature up to 100 billion degrees or more. Outer layers begin to collapse, but then rebound and are thrown violently outward with the explosion of the core.
- For days to weeks, a supernova shines extremely bright, even outshining galaxies. They spew out their elements and subatomic particles, populating the space around them. Supernova explosions occur about once every hundred years in a typical galaxy and about 25-50 supernovae are observed each year, but most are too far away to be seen without a telescope.
A star rebirth
The dust and debris strewn into space from (super)novae will slowly mix with surrounding interstellar gas and dust, enriching the area with all the heavy elements and compounds the star produced before and during its death throes. This will, over millions of years, provide the building blocks for a new generation of stars and systems, starting the process over again.
Conclusion
Just like us, stars are born, live a full life, and then die, opening the door to new life in the ashes of the fallen star. The typical life cycle of a star covers a slow timeline (compared to our own) involving the dance and later fusion of atoms until the balance can no longer be contained and explosions tear it apart, leaving behind a number of different options, many of which allow new life to form.
As we continue to study the stars with newer instruments, who knows what new data will be unearthed?

Sarah Hoffschwelle is a freelance writer who covers a combination of topics including astronomy, general science and STEM, self-development, art, and societal commentary. In the past, Sarah worked in educational nonprofits providing free-choice learning experiences for audiences ages 2-99. As a lifelong space nerd, she loves sharing the universe with others through her words. She currently writes on Medium at https://medium.com/@sarah-marie and authors self-help and children’s books.
Wow! There's more to read 🚀
This page is part of our collection of articles about astronomy. If you enjoyed the read, then you’ll love the following articles.

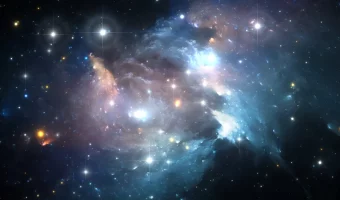
Stars are the fundamental building blocks of the universe and are responsible for creating most of the elements. What are they made of?

While there are ~1024 stars in the universe, there are less than 20 types of stars. In this article, we’ll explore star types.
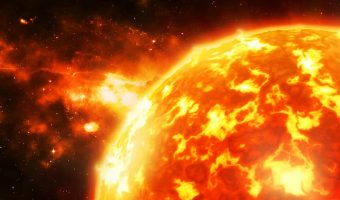
Our Sun is not a particularly large star. In fact, the universe is home to many celestial behemoths. Let’s take a look at some of the largest known stars.